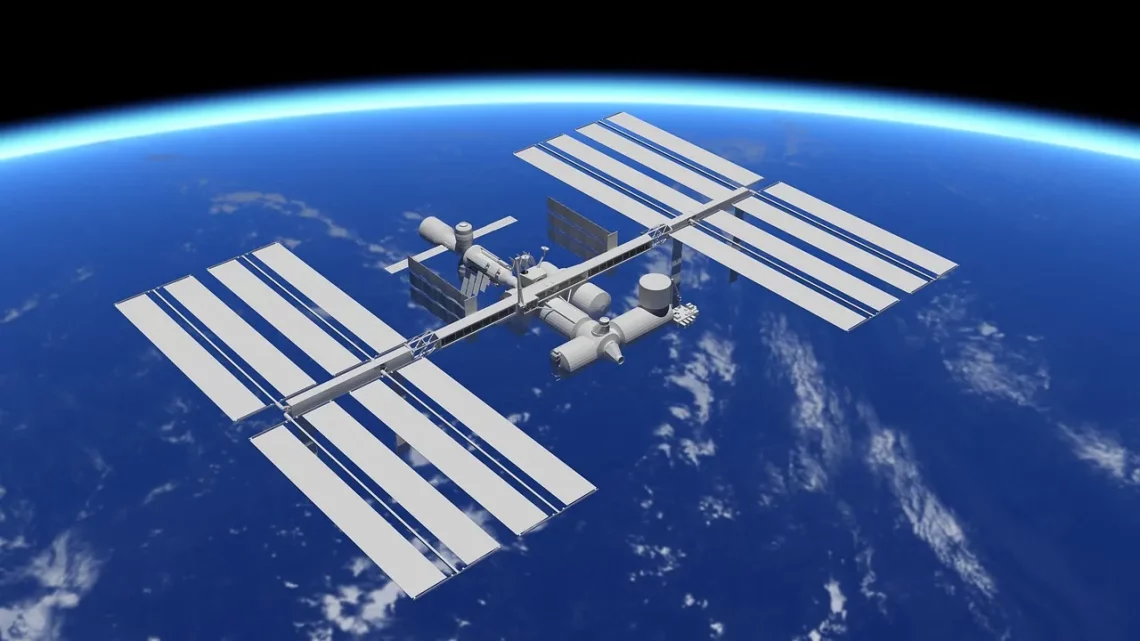
Understanding the Impact of Thruster Blast on Spacecraft Maneuverability
Space exploration has always been a subject of fascination for humanity. The vastness of the cosmos, filled with mysteries and opportunities, beckons us to explore beyond our terrestrial confines. As we venture further into space, the technology that propels us becomes increasingly sophisticated. Among these technologies, thrusters play a vital role in spacecraft maneuverability. They serve not only as engines that propel spacecraft forward but also as instruments that allow for precise movements and adjustments in the vacuum of space.
Understanding how thruster blasts impact a spacecraft’s movements is crucial for optimizing missions. The dynamics of maneuverability are complex, influenced by various factors including the type of thruster, the mass of the spacecraft, and the environmental conditions in space. As we delve into the mechanics behind these systems, it becomes evident that the interplay between thrust, control, and navigation is essential for successful space missions. The effects of thruster blasts extend beyond mere propulsion; they are integral to achieving desired trajectories, maintaining orbits, and conducting delicate maneuvers such as docking with other spacecraft or landing on celestial bodies.
In the following sections, we will explore the different types of thrusters, their operational principles, and the critical role they play in ensuring that spacecraft can navigate the vastness of space with precision and efficiency.
Types of Thrusters Used in Spacecraft
The realm of space propulsion is dominated by a variety of thruster types, each designed for specific applications and missions. The most common thruster types include chemical, electric, and hybrid systems.
Chemical thrusters are perhaps the most well-known, utilizing chemical reactions to produce thrust. These thrusters operate by burning propellant, resulting in high-pressure exhaust gases that are expelled at high speed. This type of thruster is powerful and provides a significant amount of thrust, making it suitable for launching spacecraft from Earth and for large maneuvers in space. However, chemical thrusters also have limitations, including lower efficiency and a finite amount of propellant.
On the other hand, electric thrusters, such as ion and Hall-effect thrusters, use electricity to accelerate ions or plasma. These systems are much more efficient than their chemical counterparts, allowing spacecraft to operate for extended periods with minimal propellant. Although electric thrusters produce less thrust, they are ideal for deep-space missions where high efficiency is critical.
Hybrid thrusters combine elements of both chemical and electric propulsion, aiming to maximize the benefits of both systems. By integrating chemical reactions with electric propulsion, hybrid systems can provide flexibility for various mission profiles.
The choice of thruster type directly influences a spacecraft’s maneuverability and operational capabilities. Understanding these differences is essential for mission planners to select the appropriate propulsion system based on the specific requirements of each space mission.
The Physics of Thrust and Maneuverability
At the core of spacecraft maneuverability lies the principles of physics that govern motion. When a thruster expels mass in one direction, the spacecraft experiences an equal and opposite force, according to Newton’s third law of motion. This relationship is fundamental to understanding how thrust translates into movement in the vacuum of space.
The amount of thrust generated is measured in Newtons, and its effectiveness depends on both the mass of the spacecraft and the duration of the thrust. For instance, a spacecraft with a larger mass requires more thrust to achieve the same change in velocity as a smaller spacecraft. This is where the concept of specific impulse becomes crucial. Specific impulse measures the efficiency of a propulsion system in terms of thrust produced per unit of propellant consumed.
Maneuverability is not solely determined by the amount of thrust but also by how the thrust is applied. Rapid bursts of thrust can lead to abrupt changes in velocity, which may be necessary for quick adjustments during critical phases of a mission. However, such maneuvers can also introduce risks, including structural stress on the spacecraft and challenges in stability control.
Additionally, the distribution of thrust across different thrusters can affect a spacecraft’s rotational and translational movements. By carefully managing the firing sequence and duration of thruster blasts, mission control can fine-tune a spacecraft’s trajectory and orientation. This level of control is vital during delicate operations, such as satellite deployments or planetary landings, where precision is paramount.
In summary, the physics underlying thrust and maneuverability is complex and multi-faceted. A deep understanding of these principles allows engineers and scientists to design spacecraft that can navigate the challenges of space with precision and reliability.
Real-World Applications of Thruster Technology
The impact of thruster technology on spacecraft maneuverability is evident in various real-world applications. From launching satellites into orbit to interplanetary exploration, thrusters play an indispensable role in modern space missions.
One of the most notable applications is in satellite positioning and stabilization. Satellites rely on thrusters to maintain their orbits and correct their trajectories. Over time, gravitational influences and atmospheric drag can cause satellites to drift from their intended paths. By using thrusters to perform orbital maneuvers, operators can ensure that satellites remain functional and deliver accurate data.
Another significant application is in the realm of deep-space exploration. Missions to other planets, moons, and asteroids often rely on electric thrusters for propulsion. For example, NASA’s Dawn spacecraft utilized ion propulsion to explore the asteroid belt, traveling vast distances with minimal propellant consumption. This efficiency allowed the spacecraft to enter orbit around two separate celestial bodies, providing invaluable data about their composition and history.
Additionally, thruster technology is vital for crewed missions to destinations such as the Moon or Mars. During these missions, precise maneuverability is crucial for tasks like docking with space stations or landing on planetary surfaces. The ability to control a spacecraft’s speed and trajectory can mean the difference between a successful mission and potential disaster.
As space agencies and private companies continue to push the boundaries of exploration, the development and refinement of thruster technology remain at the forefront of innovation. New advancements, such as the exploration of plasma propulsion and other emerging technologies, promise to enhance maneuverability further, making space travel safer and more efficient.
In conclusion, the role of thrusters in spacecraft maneuverability cannot be overstated. Their impact spans various applications, contributing significantly to the success of missions that expand our understanding of the universe.
Future Trends in Thruster Development
Looking ahead, the future of thruster technology is poised for significant advancements driven by the increasing demand for efficient and versatile propulsion systems. As space exploration continues to evolve, several trends are emerging that promise to enhance the capabilities of thrusters.
One prominent trend is the development of more efficient electric propulsion systems. As researchers explore new materials and technologies, we can expect to see higher specific impulse values, allowing spacecraft to operate with even less propellant. Innovations such as magnetoplasmadynamic (MPD) thrusters and advanced ion thrusters are already on the horizon, offering the potential for longer missions with reduced fuel costs.
Another trend is the miniaturization of thruster technology. As small satellites, or CubeSats, gain popularity for scientific and commercial applications, there is a growing need for compact and lightweight thrusters. Miniaturized propulsion systems can enable these small spacecraft to perform complex maneuvers and maintain their operational capabilities in challenging environments.
Furthermore, the integration of artificial intelligence (AI) in thruster control systems is an exciting development. AI could optimize thrust application based on real-time data, allowing for more precise maneuverability and decision-making during missions. This technology could enhance the safety and efficiency of spacecraft operations, particularly in complex scenarios such as autonomous landings or avoiding space debris.
Lastly, the push for sustainable space exploration is likely to influence thruster development. Researchers are exploring environmentally friendly propellants and propulsion methods that minimize the impact on space environments. As the space industry expands, ensuring that our activities do not harm celestial bodies or contribute to space debris will be a critical consideration.
In summary, the future of thruster technology is bright, with ongoing research and innovation promising to revolutionize spacecraft maneuverability. As we continue to explore the cosmos, these advancements will play a crucial role in shaping the next generation of space missions.
In conclusion, understanding the impact of thruster blasts on spacecraft maneuverability is essential for the success of space missions. The technology behind thrusters continues to evolve, driving advancements in propulsion systems that enhance our ability to navigate the complexities of space exploration. By staying informed about these developments, we can look forward to a future filled with exciting discoveries and opportunities beyond our planet.

