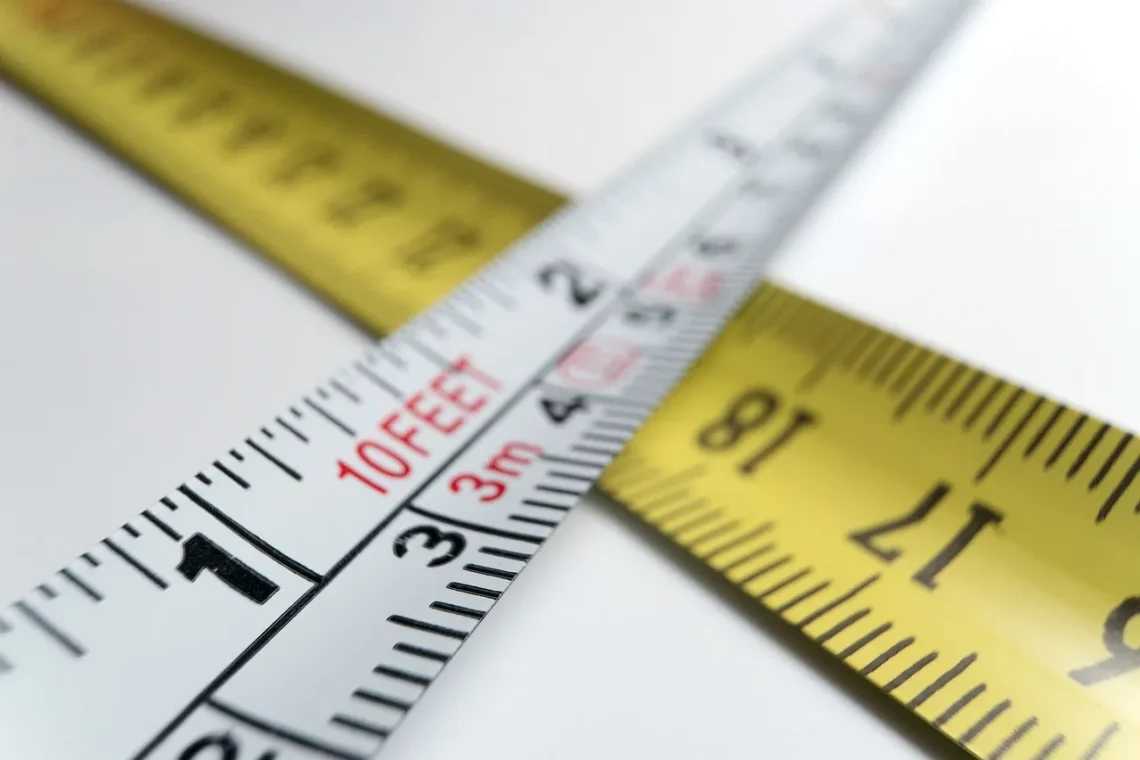
Understanding the Conversion from PM to Angstrom in Scientific Contexts
Understanding the Conversion from PM to Angstrom in Scientific Contexts
In the realm of science, precise measurements are paramount for experiments, research, and applications across various fields. With the advent of advanced technologies and methodologies, the need for accurate unit conversions has become increasingly vital. Two units that often come into play in scientific contexts are picometers (pm) and angstroms (Å). Both are used to measure extremely small distances, particularly at the atomic and molecular levels.
The picometer, which is one-trillionth of a meter, finds its utility primarily in fields such as quantum physics and nanotechnology. On the other hand, the angstrom, equal to one-tenth of a nanometer, is predominantly used in chemistry and crystallography to describe atomic-scale measurements, such as bond lengths and lattice parameters. Despite their differences in scale, understanding how to convert between these two units is essential for scientists and researchers working with nanoscale materials and phenomena.
This article will delve into the details of both units, explore their applications, and provide a clear methodology for converting between them, enhancing your understanding of these crucial measurements in scientific contexts.
Understanding Picometers and Their Applications
Picometers are a unit of length in the metric system, defined as one-trillionth of a meter (1 pm = 10^-12 m). This incredibly small scale makes picometers particularly useful for measuring distances at the atomic level. In the field of quantum mechanics, for instance, the size of atoms and the distances between their nuclei can often be described in picometers.
In chemistry, picometers are used to express bond lengths between atoms in a molecule. For example, the bond length of a covalent bond, such as the carbon-hydrogen bond in organic compounds, can typically range from 100 to 200 pm. The ability to measure and express these distances accurately is crucial for understanding molecular structures, reactivity, and the properties of materials at the nanoscale.
Another area where picometers are significant is in the analysis of crystal structures. Techniques like X-ray diffraction allow scientists to determine the arrangement of atoms in a crystal lattice, which can be measured in picometers. The data obtained helps in identifying materials and understanding their physical properties, which is essential in fields such as materials science and solid-state physics.
Moreover, advancements in nanotechnology have further highlighted the importance of picometers. As scientists manipulate materials at the atomic level, precise measurements in picometers are critical for developing new materials and technologies. For example, the synthesis of nanostructures, such as nanoparticles or nanowires, requires a thorough understanding of distances in picometers to optimize their properties for applications in electronics, medicine, and energy storage.
Thus, the picometer serves as an essential unit in many scientific disciplines, facilitating the exploration and understanding of the fundamental building blocks of matter.
Exploring Angstroms: Definition and Usage
The angstrom (Å) is a unit of length that is equal to 10^-10 meters or 100 picometers. It is primarily used in fields such as chemistry, crystallography, and solid-state physics to describe distances on the atomic scale. The angstrom is particularly beneficial when discussing atomic radii, bond lengths, and the dimensions of molecules.
One of the most common applications of angstroms is in the representation of atomic sizes and bond lengths. For example, the covalent radius of a hydrogen atom is approximately 0.53 Å, while the bond length of a water molecule (H2O) is about 1.0 Å. This level of precision is crucial for chemists, as it allows them to predict how molecules will interact based on their spatial arrangements.
In crystallography, the angstrom is used to express the parameters of crystal lattices. The arrangement of atoms in a crystal can be described in terms of lattice constants, which are often measured in angstroms. This information is vital for understanding the properties of materials, such as conductivity, magnetism, and optical characteristics.
Furthermore, in the field of molecular biology, angstrom-level measurements are essential for understanding the structure of DNA and proteins. Techniques like X-ray crystallography and nuclear magnetic resonance (NMR) rely on accurate measurements at the angstrom scale to elucidate the three-dimensional structures of biomolecules, which in turn affects their function and interactions.
The angstrom’s significance in science cannot be overstated; it provides a convenient scale for describing distances that are otherwise difficult to conceptualize. By bridging the gap between the macroscopic world and the atomic realm, the angstrom plays a pivotal role in advancing our knowledge of molecular and atomic phenomena.
Converting Between Picometers and Angstroms
Converting between picometers and angstroms is a straightforward process, thanks to the defined relationship between the two units. As previously mentioned, one angstrom is equivalent to 100 picometers. Therefore, to convert from picometers to angstroms, one must divide the number of picometers by 100. Conversely, to convert from angstroms to picometers, one simply multiplies the number of angstroms by 100.
For example, if a scientist measures a distance of 500 pm, the conversion to angstroms would be calculated as follows:
\[
500 \, \text{pm} \div 100 = 5 \, \text{Å}
\]
On the other hand, if a measurement of 2 Å is given, the conversion to picometers would be:
\[
2 \, \text{Å} \times 100 = 200 \, \text{pm}
\]
These conversions are not only useful for scientists working in various disciplines but also for students and educators who are learning about atomic and molecular measurements. Understanding how to navigate between these two units enhances clarity and precision in scientific communication.
Moreover, it is crucial to be aware of the context in which these measurements are used. While picometers may be more prevalent in quantum physics and nanotechnology, angstroms are often favored in chemistry and crystallography. Being able to convert between the two allows for more effective collaboration across different scientific fields, where a common understanding of measurements is essential.
In practice, many scientific calculators and software programs can facilitate these conversions, streamlining the process for researchers and students alike. This ease of conversion reinforces the importance of both units in scientific discourse, ensuring that precise measurements are maintained throughout various applications.
The Importance of Accurate Measurement in Science
Accurate measurement is the backbone of scientific inquiry. Whether it is measuring the length of a bond in a molecule or the distance between atoms in a crystal lattice, precision is essential for drawing valid conclusions and advancing knowledge. The conversion between units like picometers and angstroms is just one facet of the broader landscape of measurement in science.
Inaccuracies in measurement can lead to significant errors in research outcomes, potentially affecting the validity of an experiment or study. For instance, in drug development, understanding the interactions at the molecular level is crucial for creating effective pharmaceuticals. If the distances between atoms are miscalculated, it could result in ineffective or harmful compounds being developed.
Furthermore, the reliability of scientific measurements is often dependent on the instruments used. Advances in technology have led to the development of highly sophisticated tools capable of measuring at the atomic scale with remarkable accuracy. Techniques such as atomic force microscopy (AFM) and scanning tunneling microscopy (STM) provide insights into nanoscale structures, allowing scientists to visualize and manipulate materials with unprecedented precision.
The importance of standardization in measurement cannot be overlooked. The International System of Units (SI) provides a framework for maintaining consistency across scientific disciplines. Adhering to these standards ensures that measurements are universally understood and accepted, fostering collaboration and innovation on a global scale.
In conclusion, the conversion between picometers and angstroms is a fundamental aspect of scientific measurements. By grasping the significance of these units and the importance of accuracy, researchers can contribute to the advancement of science and technology, paving the way for future discoveries.
This article is intended for informational purposes only and does not constitute medical advice. For any health-related concerns or issues, please consult a qualified healthcare professional.

